Environment & Energy
Related: About this forumProcess intensification for LNG liquefaction and air separation.
I oppose the use of all dangerous fossil fuels, including dangerous "natural" gas, but I do not necessarily oppose dangerous natural gas infrastructure to the extent that it involves pipelines and liquid phase storage tanks. The reason for not opposing the fuel infrastructure as opposed to the fuel itself, is that I believe that this infrastructure can be adapted fairly well for use of the wonder fuel DME, dimethyl ether, which has a short atmospheric lifetime, v and thus, a low global warming potential when compared to the chief component of dangerous natural gas, methane.
DME is easily liquified and thus can act as a refrigerant - it has a critical temperature higher than the boiling point of water, around 128°C - and as a fuel also burns very cleanly, actually cleaner than dangerous natural gas. It can replace diesel fuel, LNG, LPG, propane, natural gas and in fact, every fluid dangerous fossil fuel now in use. It can be made via the hydrogenation of carbon dioxide. Doing so requires providing all of the energy released in its formation plus, in the case where the carbon dioxide is captured from the air or the sea, the energy loses involved in the entropy of mixing. Of course this is only possible in the case where clean energy - massive amounts of it - are available. (Clue: Wind and solar won't cut it.)
Energy efficiency is important to reduce the cost of energy to the point that those who currently lack it will have access to it, and thus it is with interest that I encountered this paper from Pakistani scientists: Conventional and Advanced Exergy Analyses of an Integrated LNG RegasificationAir Separation Process (Muhammad Haris Hamayun, Naveed Ramzan, Murid Hussain, and Muhammad Faheem) Industrial & Engineering Chemistry Research 2022 61 (7), 2843-2853.)
The bold and italics I will add to the introductory text are there to point to parts of the text of the introductory paragraph that follow are either at worst wrong, in my opinion, at best highly misleading:
LNG is a multicomponent liquid mixture with methane as the major component. Ethane, propane, butane, pentane, nitrogen, and carbon dioxide are present in smaller quantities. (3) The LNG chain involves four processes: (1) natural gas liquefaction, (2) LNG transportation, (3) LNG storage and regasification, and (4) transmission and distribution to the end-users. (9,10) LNG is imported in Pakistan via marine routes using specially designed ships. It is then regasified and injected into the existing gas pipelines for onward transmission and use.
LNG production involves cooling the natural gas to cryogenic temperatures (−162 °C, at atmospheric pressure). (11−13) The liquefaction process results in a considerable increase in energy density such that 1 m3 of LNG contains ca. 625 m3 of natural gas. (13,14) This reduction in volume is critical for the economics of LNG transportation. However, the LNG must be vaporized/regasified before injecting into the main gas distribution network. LNG-receiving terminals must ensure that the send-off gas meets the requirements of the existing gas pipeline network. This can be tricky especially when LNG is imported from different sources as the methane content in different shipments can vary from below 80% to more than 98%. (15,16) In the case of Pakistan, the gross calorific value (GCV) of the imported LNG is typically between 1050 and 1200 BTU/scf and must be lowered to between 950 and 1000 BTU/scf before the gas can be used for power generation. (17)...
The reference to dangerous natural gas being a "clean" fuel is popular nonsense, albeit one endorsed by the EU recently, probably as a dollop to dangerous fossil fuel dependent Germany. (It is an insult to the nuclear industry to place dangerous natural gas in the same category as nuclear energy with this lie about natural gas being "green."

The remark about "energy density" is also misleading. It takes lots of energy to liquify dangerous natural gas, both in the form of generating high pressures and generating low temperatures. It is fine that some of this energy may be recovered as energy for the liquefaction of air, the topic of the paper, but overall considerable quantities of energy are rejected to the atmosphere. Thus while the energy density of the already liquified dangerous natural gas may be high, the energy density of the original dangerous natural gas overall is considerably lower, vast sums of energy are wasted.
Above in my text, I emphasized the property of DME as a refrigerant. The boiling point of liquid DME is -24°C (-11°F), temperatures that can be ambient in very cold regions. The heat of vaporization of DME is 24 kg/mol, meaning that as it boils the liquid cools, something that is commonly observed in propane tanks, albeit with propane having a lower heat of vaporization (18.8 kg/mol), where sometimes one can observe ice forming on the surface of tanks in use even on a hot day. This suggests that under some circumstances one might obtain some very cold temperatures by pumping on DME, in effect, a heat pump.
The hydrogenation of carbon dioxide directly to form DME (or its intermediate methanol in some scenarios) is an exothermic reaction, and although the effect is small, hydrogen gas is unusual since it has a positive Joule Thomson coefficient; it heats when it expands. Thus during synthesis the gas can drive its own compression; the energy loss is compensated by the extreme flexibility and ease of handling, use, storage and transport of DME relative to hydrogen. Some of this lost energy in some circumstances may be usable, subject to the constraints of the second law of thermodynamics, in heat exchange networks, but ultimately DME will be liquid at ambient temperatures as a result of the so called "zeroth law of thermodynamics."
Thus there exists a potential for refrigeration using DME, and certainly one can imagine circumstances where, as is discussed in the paper referenced, for air separation.
There are many industrial and other uses for liquid nitrogen, and, of course, as became evident in the Covid crisis, medical uses for liquid oxygen. Another opportunity for oxygen as a side product is sometimes discussed in various incarnations, oxyfuel combustion.
In oxyfuel combustion, no smokestack is required, the product of the combustion is pure, or nearly pure carbon dioxide. Where the combusted product is biomass, this represents an opportunity to remove carbon dioxide from the air, otherwise an energetically expensive process, the energy required to overcome the entropy of mixing, in effect overcome by solar energy that is far less pernicious than PV cells and/or (worse) thermal solar schemes.
Thus this process intensification described in the referenced paper has some value for consideration, even if it involves dangerous natural gas as opposed to something like DME.
I note for the short term, owing to the adventures of the criminal Vladimir Putin, there will be more LNG used rather than less, with more energy wasted, more climate effects of the already unacceptable effects of dangerous natural gas waste, particularly in places where clean infrastructure was willfully destroyed, for example Germany. If some of the exergy of this sad and avoidable situation can be recovered, well, so much the better.
Some text referring to what the devices in the flow charts below represent:
...Both the air streams leaving the compression section (S114 and S115) enter the cryogenic section. They are first cooled in MHEX E104, using the products (S127 and S129) and side-stream (S132) coming from the LP column (C102). The cooled air streams are then throttled (valves V101 and V102) to further reduce their temperature and fed to the HP column (C101). The products leaving the HP column (S120 and S121) are further cooled in the second MHEX (E105) using the top product of the LP column (S126). They are then throttled (valves V103 and V104) and fed to the LP column (C102). High-purity nitrogen (99.1%) is obtained from the top of the LP column (S126). High-purity oxygen (99.1%) is obtained from the bottom of the LP column (S129). Two side-streams (S131A and S131B) are withdrawn from stages 8 and 46, respectively, to remove undesired argon from the system and maintain the purity of the nitrogen and oxygen products. The cold energy of all product- and side-streams leaving the LP column is recovered in MHEXs E104 and E105. Both columns are heat-integrated (HI) such that the duty of the condenser of C101 is equal to the duty of the reboiler of C102. The operational conditions of the standalone ASU are presented in Table S1
Some text, as a graphics object to preserve subscripts and superscripts giving insight to what they mean.:
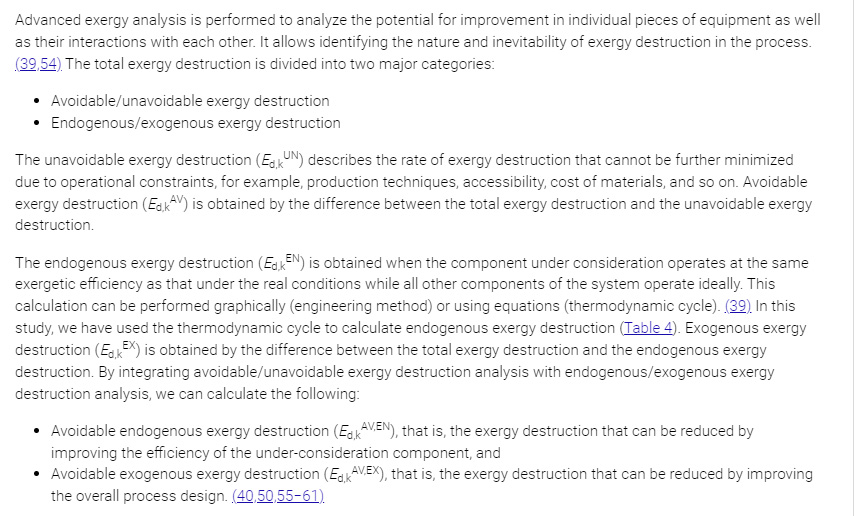
A table in the paper may also explain to what some of the symbols in the graphics below following apply:
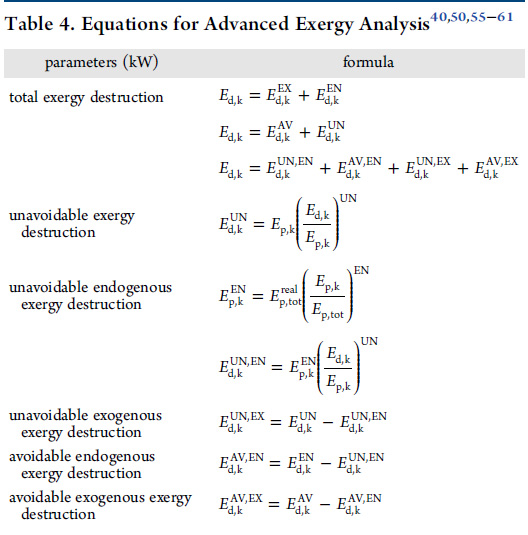
Some graphics from the paper:
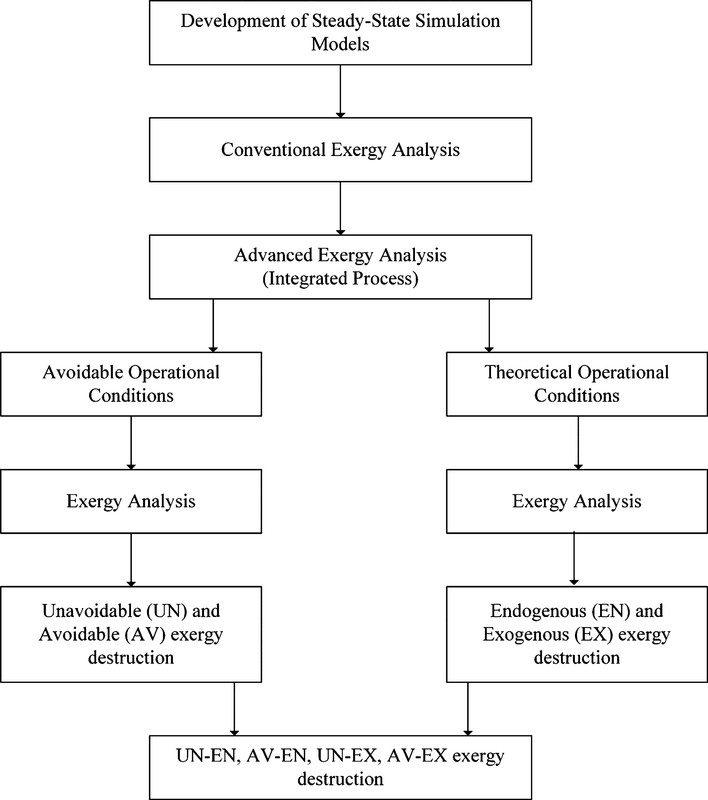
The caption:
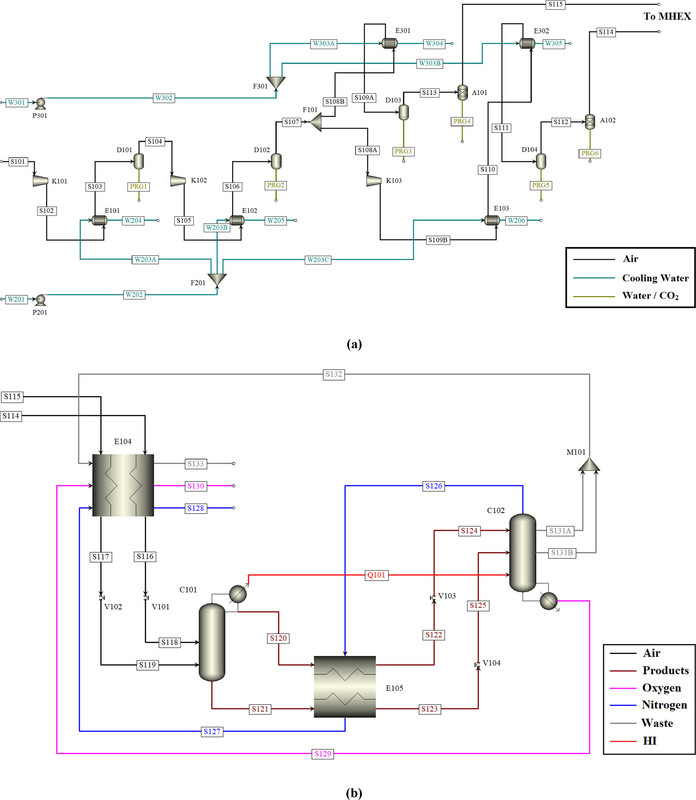
The caption:
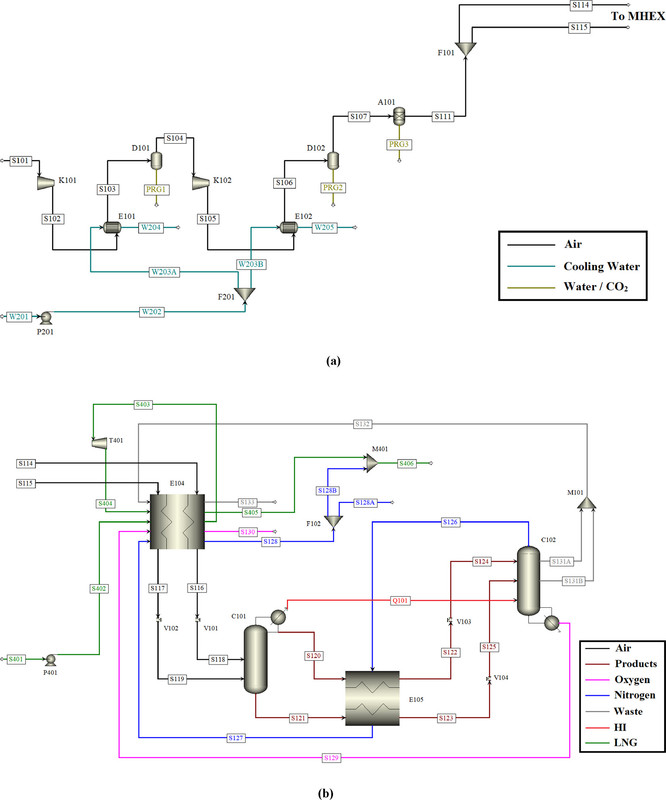
The caption:
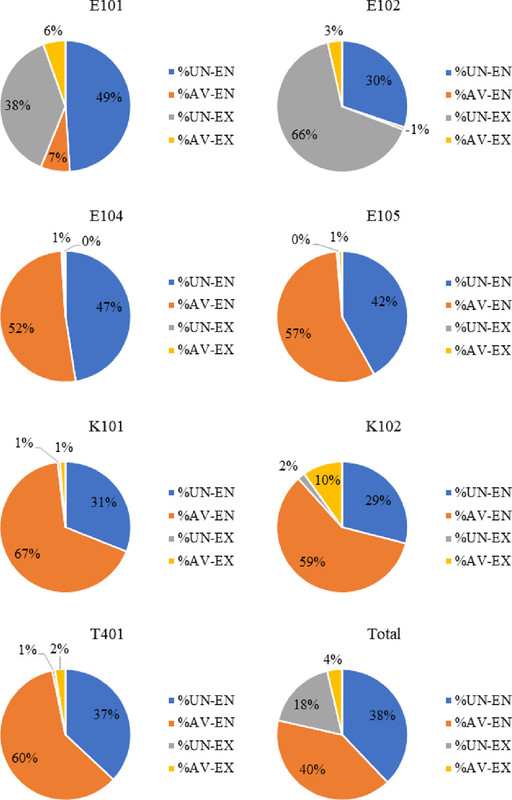
The caption:
From the conclusions:
Only 12.6% exergy destruction in the first water-cooled heat exchanger (E101) is avoidable. However, the endogenous and exogenous components are nearly equal, showing that both the equipment efficiency and the external interactions are important for the reduction in exergy destruction.
The exergy destruction in the second water-cooled heat exchanger (E102) is largely unavoidable. Furthermore, the avoidable exergy destruction is largely of exogenous nature, and improving the performance of the upstream compressor (K102) is the only way to reduce exergy destruction in this exchanger.
The key to future of humanity, including that of extending the benefits of energy to those who lack it as well as well as to cleaning up the mess we made, particularly of the atmosphere, but also of every body of water on the planet, is high efficiency, in addition to ignoring antis who demonize nuclear energy. (The purpose of efficiency is not to reduce energy use, but rather to expand its availability as human a justice issue.)
I trust you're having the best weekend possible in a time of tragedy.