Science
Related: About this forumExploration of the Plutonium/Plutonium Hydride Phase Diagram.
Last edited Sun Jan 31, 2021, 02:28 AM - Edit history (1)
(Graphics in this and previous posts may not be visible in Google Chrome, but should show up in Microsoft Edge, Firefox and Android.)
The paper I'll discuss in this post is this one: Quantum Accurate Prediction of PlutoniumPlutonium Dihydride Phase Equilibrium Using a Lattice Gas Model (Ryan Gotchy Mullen and Nir Goldman, The Journal of Physical Chemistry C 2020 124 (38), 20881-20888).
Not so long ago in this space, I noted that in nuclear fission, some americium isotopes exhibit a very high yield of neutrons, called generally "multiplicity," and thus are capable of extraordinary breeding ratios: Electrochemical oxidation of 243Am(III) in nitric acid by a terpyridyl-derivatized electrode.
Although americium will always be produced in a fission nuclear reactor having any kind of fuel efficient high burn up, the reality is that, even though Am-241, the lightest commonly available americium isotope, exhibits high multiplicity in the fast neutron spectrum, it is still 3 mass units separated from its natural source, uranium-238. It is also true that there is not enough americium on the planet - even though inventories in used nuclear fuel are significant - to work toward the immediate elimination of dangerous fossil fuels while still preserving human development goals and the elimination of poverty.
There is, by contrast, enough plutonium available in used nuclear fuel to power all of the world's energy demand, roughly 600 EJ/year, by utilizing a type of nuclear reactor currently under commercial development by a number of companies and laboratories: The "Breed and Burn" type reactor, Sekimoto's "Candle Reactor" and variants thereof. This strategy would be sufficient to provide all of humanity's energy needs for centuries without operating a single energy mine of any kind anywhere on the planet using uranium already mined and isolated - including "depleted uranium" - as well as the thorium mined and dumped in order to serve the ridiculous so called "renewable" wind industry and other lanthanide dependent industries.
Light A Candle, An Innovative Burnup Strategy for Nuclear Reactors.
In order to assure that sufficient plutonium remains available for this purpose, as well as to provide for other uses for neutrons beyond fuel breeding, it is desirable to have as high a multiplicity as possible for plutonium. In the fast neutron spectrum, plutonium is always a breeder fuel, but how well it breeds is a function of the chemical form of the element, for example, whether it exist as oxides or nitrides or some other compound. Metallic plutonium, although it has a complex phase diagram, gives the highest multiplicity among these options. Very old literature, from the 1960's, indicates that the highest multiplicity ever observed in plutonium, giving a breeding ratio of over 1.5, is available in liquid plutonium. (cf. E.V. Evans, Editor, Fast Breeder Reactors, Proceedings of the London Conference on Fast Breeder Reactors, 17-19 May, 1966, Whitman, in "Fast Breeder Reactor Development in the United States, paper 3/2 pg. 286 )
I have been thinking about and studying the literature associated with liquid plutonium for a number of years now with particular attention to an experimental reactor that was being described in the 1966 reference just above, which operated at Los Alamos nearly sixty years ago, the LAMPRE reactor. This reactor utilized tantalum capsules to contain the plutonium, tantalum and tungsten being the only two metals that do not dissolve in liquid plutonium. Tantalum was selected because of the difficulty of machining and welding tungsten.
No device dependent on tantalum can be considered sustainable, since the element is a "critical element," one which is easily subject to depletion with rising use. In addition, it is a "conflict element," an element mined under appalling conditions often involving human slavery, where some of the slaves are children. (The main use for the element today is for supercapacitors in cell phones.)
It does seem to me that advances in materials science over the last 60 years may well eliminate the need for tantalum for the purpose for liquid plutonium, and that composite ceramics may be the key to solving this technical problem.
During operations of LAMPRE, it was noted that the surface of the liquid plutonium during operation became coated with a solid material. To my knowledge it was not chemically analyzed, but most likely it was metallic and contained fission products like the element strontium (which is insoluble in liquid plutonium and has a melting point of 777°C, higher than the LAMPRE operating temperature) as well as intermetallic phases like those between zirconium, a fission product, and plutonium.
(Plutonium Handbook, O.J. Wick Ed., M.D. Freshly, Vol 2, Chap 20 . pg 662-664, Gordon and Breach Scientific Publishers 1967. This offers a fairly illuminating, if brief, description of the irradiation of a liquid plutonium/iron eutectic in the LAMPRE reactor.)
Here is the phase binary diagram of the zirconium-plutonium system:
(cf. Plutonium Handbook, O.J. Wick Ed. Ellinger, Land and Gschneidner, Vol 1, Chap7. pg 227, Gordon and Breach Scientific Publishers 1967)
Although this binary phase diagram comes from a reference now 53 years old, it is essentially equivalent to the ATSM database version published in 2007 which includes more recent references, with the benefit that this older version is better labeled with allotropes as opposed to more arcane space groups accessed by keys in the ASM version. It is notable that the ASM version shows more completely the closing of the ellipsoid Zr solid + Pu liquid region at increasing Zr concentrations up to the melting point of zirconium, 1855°C, as one should well expect.
An aptly named review article from last year refers to this phase diagram as well as CALPHAD (Computer Coupling of Phase Diagrams and Thermochemistry) models and other updates: Experimental and Modeling Review of the Plutonium-Zirconium (Pu-Zr) System: Lost in Translation and Over Time? (Aurélien Perron & Patrice E. A. Turchi , ( J. Phase Equilib. Diffus. 41, 756763 (2020))
In any case, this binary diagram is a vast over simplification of the putative nature of the solid phase on the LAMPRE fuel surface, but does indicate that it is possible to saturate liquid plutonium with zirconium. I have in my files, more complex ternary phase diagrams, for example, the iron zirconium plutonium system and the iron uranium zirconium system, but I will spare the reader these. Real systems are even more complex, given the plethora of elements in the periodic table present as fission products and/or structural materials.
Nevertheless, these complex systems suggest the possibility of peritectoid systems, in which solid phases crystallize out of solutions. It is a property of peritectics that on occasion they can represent inert coatings, since they are quasi-stable at the peritectoid point, with limitations in further penetrations of a given element into this system, something that was not likely explored very deeply half a century ago.
Although historically it was believed that compound formation was not involved in these phases, it seems that the modern interpretation is quite different and that several of the phases are indeed thought to be peritectoid in nature.
References 4 and 15, however, date from the 1960's.
Nevertheless one can look at something approximating a "synthetic peritectoid" of plutonium to moderate the rate of corrosion in the design of a nuclear reactor that is in fact, designed to eat through its fuel. Modern computational capabilities, not available in the period between 1960-1980 -when the bulk of our nuclear fleet much of which still operates today was designed and built - can certainly play a role in materials design that must be employed to allow nuclear energy to do what it must do to save the world from the ongoing disaster of climate change.
Thus examination of the phase diagrams of plutonium with other elements always catch my eye. Hydrogen is moderating, of course, which precludes a "breed and burn" system, so it's not clear that this particular system would be useful as a containment strategy exploiting the benefits of liquid plutonium. But it is the process, and not the result, which is most important here.
From the introduction to the text:
The complexities of plutonium hydriding can be illustrated by a comparison to hydriding in a more common metal, such as palladium. The critical temperature of the palladiumpalladium hydride phase envelope is 550 K (277 °C), well below the palladium metal melting temperature of 1828 K (1555 °C).(4) In contrast, solid plutonium dihydride (PuH2) will crystallize from liquid Pu that is exposed to hydrogen gas.(5) In addition, hydriding in face-centered cubic (fcc) δ-Pu (of interest for engineering applications because of its high ductility(6)) induces a large volume expansion of 54%, compared to an expansion of only ∼10% in palladium hydriding, resulting in flaking and the complete degradation of Pu-based materials because of this lattice mismatch. Finally, neutron diffraction reveals that H atoms randomly occupy interstitial octahedral (O) sites in palladium hydride.(7) In contrast, no neutron diffraction studies have been performed on δ-Pu or PuH2 to indicate how H atoms partition between O sites and tetrahedral (T) sites, though investigations of lanthanide hydrides show that O sites are not occupied until all available T sites are filled.(8)
Conducting experiments with plutonium is exceedingly difficult because of its toxicity and radioactivity. The lowest temperature at which the composition of the PuH2 phase has been reported is 773 K (500 °C).(5) Mulford and Sturdy additionally reported equilibrium pressures at 673 and 723 K (400 and 450 °C) but were unable to determine the equilibrium compositions at these temperatures, reporting that it took 20 h to equilibrate at each trial composition...
I have always known that plutonium is a very active metal, but the idea that hydrides are involved in the dissociative absorption of water into the metal is something I had not considered. This should have some implications in connection with the long term stability of nuclear weapons, reducing their reliability - a good thing - I would think if the core is not desiccated with regular maintenance to address saturation of the desiccant would further reduce their viability of weapons. (We need to do a "sword into ploughshares" kind of thing with plutonium, something with which VP Al Gore had notable success with negotiating with Russia in the 1990's with enriched uranium, pre-Putin of course.)
Later on, the introduction gives the paper's raison dêtre:
Ga-stabilized δ-Pu is the form of plutonium utilized in nuclear weapons. An interesting and fun bit of history is that the Russians and the Americans each figured out in the 1950's that they both were using this alloy in their weapons because the gallium/plutonium alloy was the only phase diagram that was consistently not published in the open scientific literature because it was regarded as "classified."
The paper goes on to discuss the issue of computer time required to do various types of calculations associated with computer models, density function theory (DFT) for example and lattice gas models. On a simplistic level, these calculations should be expected to be computationally expensive given the fact that plutonium metal involves 94 electrons for each atom, but great strides have been made in the last 50 years in accessing meaningful data using approximations, a task that was initiated, among other places, with the Born-Oppenheimer approximation which assumed static nuclei. People with a college level background in quantum physics/chemistry will recall that exact solutions to energy calculations only exist for the (unbonded) hydrogen atom, and all other calculations in all other systems require iterative calculations that converge on solutions. These systems have become increasingly sophisticated and useful. Just one example is Orbital-free density functional theory which is an "electron gas" model for finding the solutions which the Kohn-Sham theorem predicts.
In the text a simplification utilized is to restrict hydrogen atoms in plutonium hydride to tetrahedral (T) and octahedral (O) sites in the lattice.
Some sample math porn from the paper:
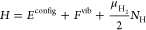
where the configurational potential energy Econfig, the vibrational free energy Fvib, and number of hydrogen atoms NH are properties of the microstate while the chemical potential of a gas-phase hydrogen molecule μH2, the number of plutonium atoms NPu, and the temperature T are held constant. Each absorbed H is treated as an independent quantum harmonic oscillator. Consequently, eq 1 contains the analytic free energy Fvib rather than a potential energy Evib and corresponding quantum degrees of freedom. A PΔV term for H in the Pu lattice is not included in eq 1 because under the conditions studied here, its value is several orders of magnitude smaller than other terms. The impact of plutonium defects on PuPuH2 equilibrium is not considered in this work...
...Because of the likely strong screening effects of the intervening plutonium atoms, we parameterize the change in energy using only the number of occupied nearest neighbor sites surrounding an occupied O or T site. This results in three types of HH structures: those comprised of hydrogens in neighboring T sites (ETT), those in neighboring O sites (EOO), and cross-interactions (EOT). As will be shown, all OO and OT structures are sufficiently energetically prohibitive as to be rare in either δ-Pu or PuH2 phases. Accordingly, we ignore the change in energy induced by an OO structure when OT structures are present because the latter increase the energy significantly more than the former. The configurational potential energy is

where ni is 1 if site i is occupied and 0 otherwise, and the function EOT accounts for HH interactions between a hydrogen in an O site and the NiT hydrogens in the nearest neighbor T sites. ETT, EOO, and NiO are defined similarly. The number of occupied neighbors NiT and NiO are computed using periodic boundary conditions. The first sum is over T sites, the second sum is over O sites, and the Heaviside function θ (x) is 1 if x ≥ 0 and 0 otherwise.
Zero-point energies and entropic contributions from the vibration of light interstitials such as H in metals can be significant. Changes in the plutonium vibrational frequencies are not included because Pu atoms are much heavier than the absorbed H atoms. The insertion of a hydrogen atom, on the other hand, creates three vibrational modes that did not exist in the previous configuration. These modes are treated as quantum harmonic oscillators, with the following analytical free-energy expression
where ni is 1 if site i is occupied and 0 otherwise, and the function EOT accounts for HH interactions between a hydrogen in an O site and the NiT hydrogens in the nearest neighbor T sites. ETT, EOO, and NiO are defined similarly. The number of occupied neighbors NiT and NiO are computed using periodic boundary conditions. The first sum is over T sites, the second sum is over O sites, and the Heaviside function θ (x) is 1 if x ≥ 0 and 0 otherwise.
Zero-point energies and entropic contributions from the vibration of light interstitials such as H in metals can be significant. Changes in the plutonium vibrational frequencies are not included because Pu atoms are much heavier than the absorbed H atoms. The insertion of a hydrogen atom, on the other hand, creates three vibrational modes that did not exist in the previous configuration. These modes are treated as quantum harmonic oscillators, with the following analytical free-energy expression

Here, Θij is the vibrational temperature ℏωij/kB for frequency ωij of a hydrogen in site i along normal mode j. We parameterized Θij as a function of NiT to account for the decrease in ωij as the plutonium lattice expands with the absorption of hydrogen...
Some pictures from the text:
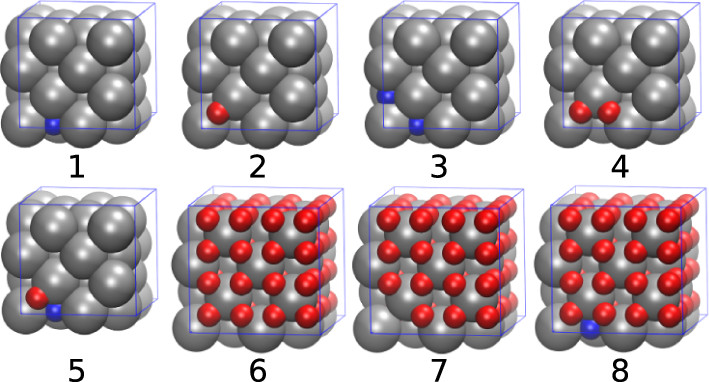
The caption:
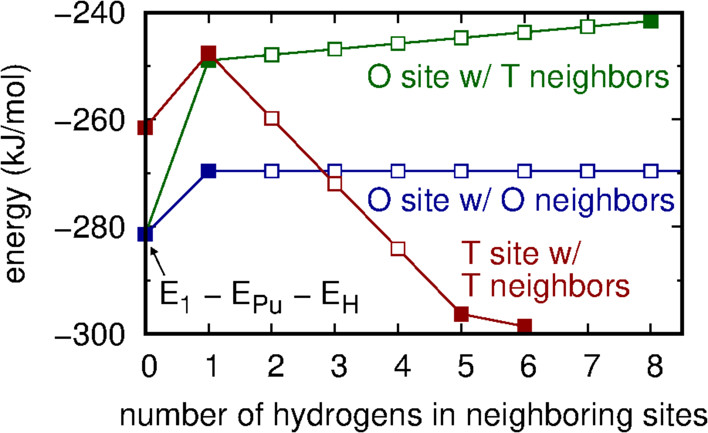
The caption:
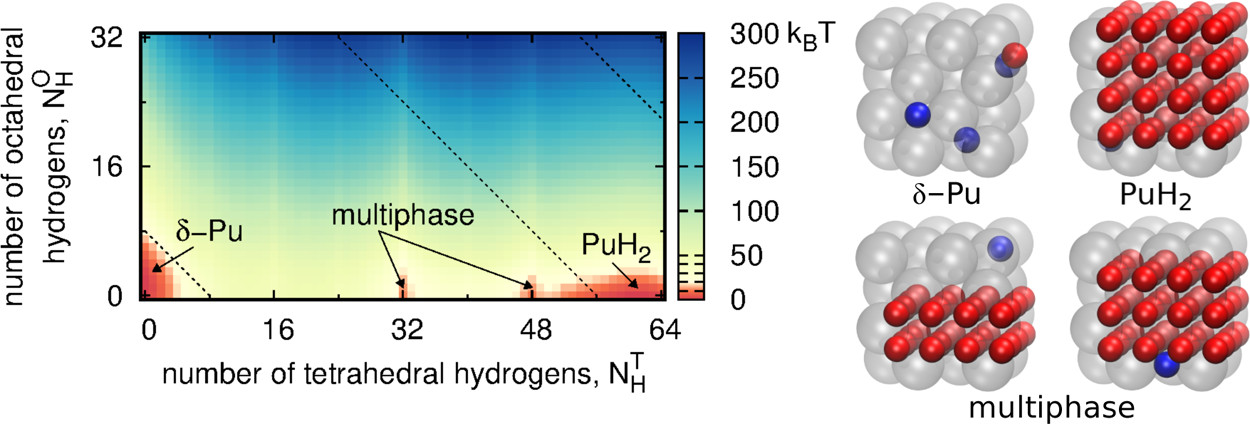
The caption:
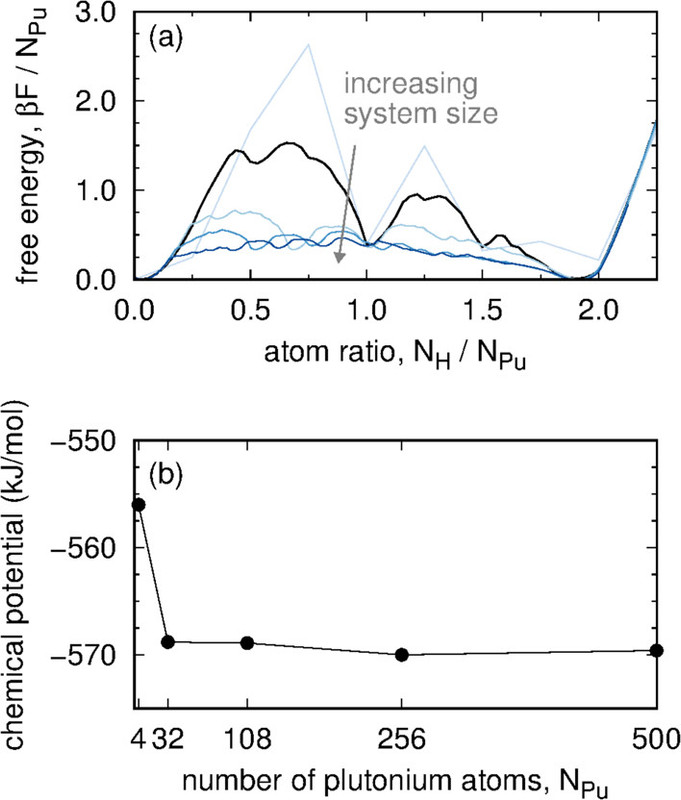
The caption:
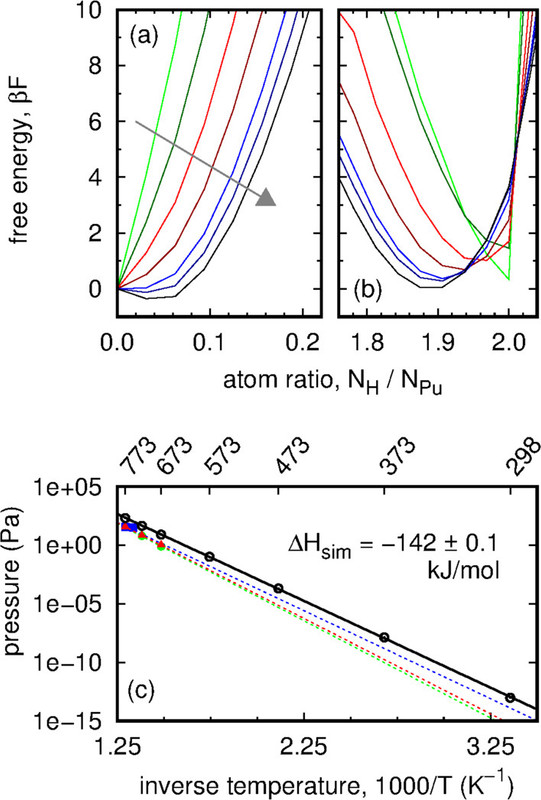
The caption:
From the conclusion to the paper:
...We anticipate that our lattice gas model could be parameterized to examine the hydride phase envelopes of other fcc actinides, rare earth metals, or transition metals (e.g., thorium, cerium, and palladium). Overall, our predictions provide a baseline to guide future plutonium experiments...
I'm not shy in offering my opinion that if we are to save the world, we should definitely have lots and lots of "future plutonium experiments"
I trust you're having a nice and safe weekend, as we all look forward to having a real President of the United States again in this coming week.